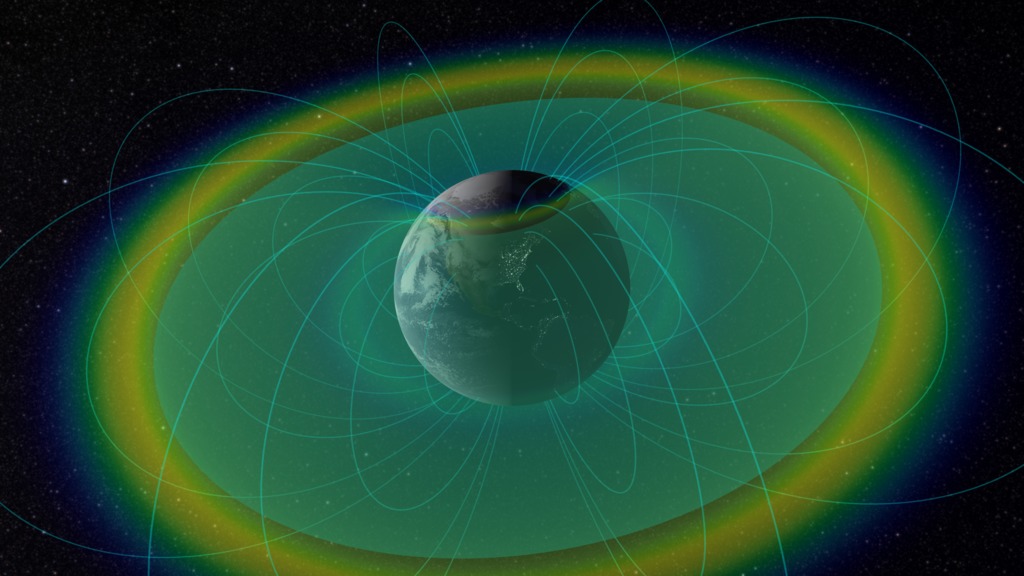
NASA/Goddard/Scientific Visualization Studio
Earlier this week, millions of Americans on Earth, as well as those living off it, came together to celebrate Thanksgiving—a time-honored holiday tradition of expressing gratitude for life’s blessings. Yet one thing that everyone on Earth should be thankful for is the protection offered by a nearly impenetrable barrier in space that was recently discovered inside our planet’s radiation belts, which prevents all the very high-energy electrons that are trapped inside them from reaching the surface and pose a threat to life.
Coined as the first scientific discovery of the Space Age, the existence of the radiation belts that surround our planet was revealed by the first U.S. artificial satellite Explorer 1 in early 1958, and subsequently by Explorer 3 and Pioneer 3 later that same year. These belts, named after the American space physicist James Van Allen who first detected them, consist of mainly two donut-shaped regions inside which high-energy, fast-moving particles are trapped by the Earth’s magnetic field, whipping around our planet at great speeds of many thousands of km per second. The first region that is closest to Earth, called the “inner” belt, extends from an altitude of about 600 km to 6,000 km (0.2 to 2 Earth radii) above the surface and contains mainly very high-energy protons in the range between 10 and 100 MeV. The second region, called the “outer” belt, lies much farther out, extending between 13,000 and 65,000 km (three to 10 Earth radii) above the surface, and contains a population of lower-energy electrons and various ions in the range between 10 KeV and 1 MeV that rises to higher energies only during powerful geomagnetic storms, as a result of solar flares, coronal mass ejections, and other energetic phenomena on the Sun which cause disturbances in the Earth’s magnetic field.
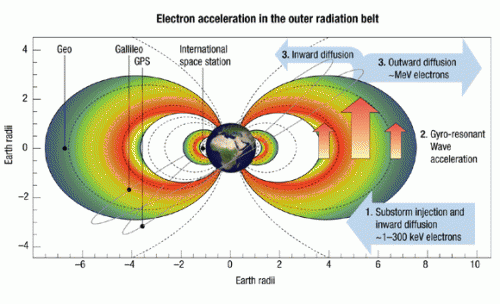
For many years after they were discovered, the Van Allen radiation belts were thought to be somewhat static and unchanging. Various space missions which have studied the Earth’s wider magnetic environment in the following decades revealed a different picture, however, showing that the belts were quite dynamic, continuously changing in size and intensity in ways that were poorly understood. Furthermore, our modern civilization’s ever-growing dependence on space assets for many essential every-day activities, like telecommunications, weather forecasting, and terrestrial navigation, underscored the importance of better understanding the physics behind all the high-energy processes that take place within the our planet’s radiation belts, which can disrupt the normal operation of our orbiting satellites and can also pose a serious threat to the health of human crews on space missions to low-Earth orbit and beyond.
To that end, NASA launched the Van Allen probes mission, consisting of two identical spacecraft (formerly known as Radiation Belt Storm Probes, or RBSP) in August 2012 as part of the agency’s Living with a Star program, with the goal of better understanding and ultimately predicting the behavior of the Earth’s magnetic field radiation environment, by allowing scientists to study how the Sun influences space weather. The major difference of this mission is that where most spacecraft try to avoid the harsh radiation environment of the belts, the Van Allen probes aimed straight for them in order to obtain direct measurements of their radiation flux. Placed into a highly elliptical 600 km by 30,000 km, 9-hour-period orbit over the equator where the belts are most intense, the twin spacecraft cover the entire radiation belt region while speeding through it at more than 3,000 kph. While still in the early stages of their commissioning period, the probes made their first major discovery within just two days following launch by revealing the existence of a third, transient radiation belt—a completely unexpected finding. In addition, the mission also helped to establish that the belts act as natural particle accelerators and allowed scientists to improve on their theoretical models of space weather. Now in a new study which appeared in the Nov. 27 issue of the journal Nature, a U.S. research team, led by Dr. Dan Baker, director of the Laboratory for Atmospheric and Space Physics at the University of Colorado, presented the mission’s latest findings of an extremely sharp boundary at the inner edge of the outer Van Allen belt, at an altitude of approximately 11,000 km above the Earth’s surface, which acts as an invisible barrier that prevents all the highest-energy electrons that swirl around in our planet’s magnetic field lines from reaching the surface.
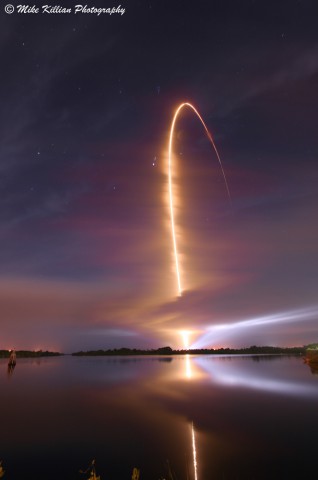
The surprising discovery was made following an analysis of data that were gathered by the Relativistic Electron Proton Telescope, or REPT, onboard the twin Van Allen probes during their first 20 months of operations, between September 2012 and May 2014. More specifically, the researchers observed that during that time nearly all of the high-energy electron flux inside the outer radiation belt never approached our planet to a distance below 2.8 times the Earth radii from the surface, even at times of heightened solar activity. “The operational period of the Van Allen probes mission has been relatively quiet geomagnetically, and the plasmasphere region (the inner part of the Earth’s magnetosphere), often extended outward to 5 times the Earth radii or further,” write the researchers in the study. “Thus, contrary to prior expectations, the inner edge of the highly relativistic electron population measured by the REPT instrument on board each of the two spacecraft, was rarely collocated with the plasmapause (the outer boundary of the plasmasphere). Instead, an almost complete lack of very high-energy electrons (in a region of slot morphology) was seen only (but persistently) for distances beyond 2.8 times the Earth radii … The inner boundary of ultra-relativistic (7.2 MeV) electron trapping was extremely sharp and stable for many months. Even when external solar wind driver events cause erosion of some part of the higher altitude population … the ultrarelativistic electrons remained persistently high in intensity at distances beyond 2.8 times the Earth radii and showed no measurable flux at lower altitudes.”
“When you look at really energetic electrons, they can only come to within a certain distance from Earth,” says Shrikanth Kanekal, deputy mission scientist for the Van Allen Probes at NASA’s Goddard Space Flight Center in Greenbelt, Md., and co-author of the study. “This is completely new. We certainly didn’t expect that.”
Baker’s team examined several proposed mechanisms that could generate such an electron barrier in the radiation belts, like the transmission of very low-frequency radio waves from man-made activities, or other natural processes that were associated with the way that the Earth’s magnetic field was shaped, ultimately rejecting all of them. “It’s almost like theses electrons are running into a glass wall in space,” said Baker in a press release. “Somewhat like the shields created by force fields on Star Trek that were used to repel alien weapons, we are seeing an invisible shield blocking these electrons. It’s an extremely puzzling phenomenon.” The concluding hypothesis among Baker’s team is that those high-energy electrons are probably scattered away from Earth by our planet’s own plasmasphere—a region of cold ionised gas that extends from the outer edges of the ionosphere at an altitude of about 600 km all the way to the inner edges of the outer belt where the electron barrier was discovered. “The scattering due to the plasmapause is strong enough to create a wall at the inner edge of the outer Van Allen Belt,” says Baker. “But a strong solar wind event causes the plasmasphere boundary to move inward.”
In order to learn more about these latest findings, AmericaSpace contacted deputy mission scientist for the Van Allen probes Mr. Shrikanth Kanekal and asked him a few questions about the nature of the newly discovered electron barrier, as well as the implications of some of the mission’s findings to other areas of space science and exploration.
AmericaSpace: Mr. Kanekal, I’d like to thank you for taking the time to answer some questions for our readers. Would you like to give us more details about the mission’s latest discovery?
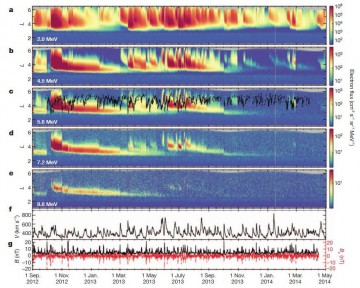
Shrikanth Kanekal: Sure. If you look at our Nature paper, what we see is that the outer Van Allen belt is mostly composed of electrons of varying energies. As we look at the highest energy electrons, they don’t seem to cross a certain distance as measured from going outward from the Earth. So in terms of Earth radii, they never seem to come below 2.8 times the Earth radii. So there seems to be some ‘barrier’ for the very energetic electrons, which seems to prevent them from coming any closer to Earth, unless there is a very strong compression event caused by coronal mass ejections from the Sun. This seems to be a naturally occurring barrier, beyond which those electrons cannot come closer to the Earth.
AmericaSpace: Are there any hypotheses to explain the mechanisms that drive this phenomenon, and was it something that was predicted by theroretical models, or was it something completely unexpected instead?
Shrikanth Kanekal: I would say it was more of the latter. It was sort of unexpected, sort of an experimental discovery from observational results, if you will. Now that we have detected it, we can speculate as to what could be possibly causing it from a theoretical perspective. And what we think is happening is that as the electrons are energized, they move closer to the Earth in a process called ‘radial diffusion’ or ‘radial discharge.’ During this process, they are also scattered and removed from the radiation belts and dumped into the atmosphere. So we think that there is a balance between the slow inward motion of these particles and their removal through this scattering process. So the net effect is like having a wall that prevents them to come further inward.
AmericaSpace: Based on the mission’s findings about the structure of the Earth’s radiation belts, do we find that it is similar to that of the radiation belts that surround the gas giant planets in our Solar System, like Jupiter and Saturn, or are there completely different?
Shrikanth Kanekal: I’m not an expert on other planets, but I have studied high-electron physics and I believe that the magnetospheric processes that are occurring on Earth are sort of universal. They have an applicability to other planetary magnetospheres and perhaps even have a similar astrophysical context like nebulae and so forth. I’m by no means an expert on the latter, but if you look at our science paper we cite some experts especially on the astrophysical contexts. If you check those out, you can see that some aspects of the magnetospheric physics that we study close to the Earth is applicable in an almost universal setting wherever there are these kinds of magnetic fields.
AmericaSpace: So in essence, the Van Allen probes mission can help us to not only study the magnetospheric processes inside the Earth’s radiation belts, but they can also help us to understand similar processes on very distant planetary and astrophysical objects as well?
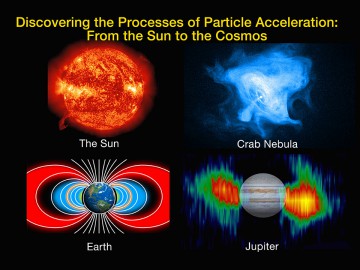
Shrikanth Kanekal: Yes. The Earth itself provides an easily accessible laboratory, if you will, because it is much more expensive to send a probe to Jupiter than to send a probe to study near-Earth space. The Earth’s magnetosphere is like a cosmic laboratory where we can study phenomena which are applicable in many other astrophysical and planetary contexts as well.
AmericaSpace: The third radiation belt that the Van Allen probes discovered last year was found to contract and expand while responding to the intensity of the Sun’s activity. Is the newly discovered electron barrier in the belts also influenced by the effects of space weather as well, or does it have a more stable structure?
Shrikanth Kanekal: In order for the more energetic electrons inside the belts to be affected by space weather, the event has to be very powerful and extreme. In that case, the electrons can break this barrier and come closer. So that has to be a very strong, energetic event, similar to the one that had been observed by the Combined Release and Radiation Effects Satellite in March 1991, where much more energetic electrons that what we have seen with the Van Allen probes, were observed to come much closer to the Earth.
AmericaSpace: What kind of danger would such a powerful event pose to our space assets, like our orbiting satellites? Can the radiation shielding that has been used on the Van Allen probes mission also be applied to other spacecraft bound for low-Earth orbit or other deep-space destinations?
Shrikanth Kanekal: In principle yes, but every mission is kind of unique. For example, interstellar space has a different kind of radiation like very high-energy cosmic rays and so forth. Speaking of the Earth’s radiation belts, what we can do is if we’re able to really understand the physics that drive them and if we’re able to predict what conditions give rise to their very energetic events, then perhaps the satellites can be maneuvered in such a way that they can escape the most intense part of the radiation.
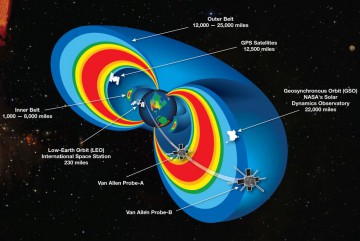
If these very energetic electrons remain in very high flux levels for a significant amount of time, then they may cause a breakdown on the circuitry of satellites by a process called ‘deep dielectric discharge.’ For example, our modern society depends a lot on satellites for GPS navigation, cellphone communications, and so forth. There is a danger that some of these satellites can be affected, resulting in the loss of telecommunications and satellite-based navigation. Those kinds of impacts could affect us much more today, because our human society depends so much more on satellites.
As to the kind of radiation shielding, the instruments on board the Van Allen probes that discovered this impenetrable barrier in the outer radiation belt were designed with lots of shielding around it so that we would be able to detect electrons only from the instruments’ field of view and not from everywhere else because we needed to measure them properly in order to better understand where they are coming from, what their energy is, etc. So that type of shielding can be done for similar spacecraft instrumentation, now that we have a history of measurements in the radiation belts on equatorial orbits where the electron fluxes are most intense.
AmericaSpace: Mr. Kanekal, I’d like to once again thank you for your time and wish you all the best of success in your research!
Shrikanth Kanekal: Thank you as well!
As is often the case, space missions hold many multi-faceted benefits for various different aspects of humanity’s life on Earth as well as in space. Ultimately, the knowledge obtained from the Van Allen probes mission will help scientists to better forecast space weather which in turn will help them develop new technologies in order to avoid the very real threat of a severe space weather event crippling our modern-day dependence on space-based technology, In addition, a better understanding of the physics that drive space weather could also enable the design of better radiation shielding techniques for protecting astronauts who will again travel beyond the Earth’s protective magnetic field in the near future on exploratory missions to deep space.
– Article written by both Leonidas Papadopoulos and Mike Killian.
Want to keep up-to-date with all things space? Be sure to “Like” AmericaSpace on Facebook and follow us on Twitter: @AmericaSpace
It is amazing to know.Iwish if could be possible by the probes to get data from extragalactic planets similar to our earth. about 34oLY away from our solar system.However at preliminary stage it requires resemblance but the Conditions will be varying and will require anti electron particles barrier there at.since theory of existance of anti events’ supplement to string theory permits accordingly.