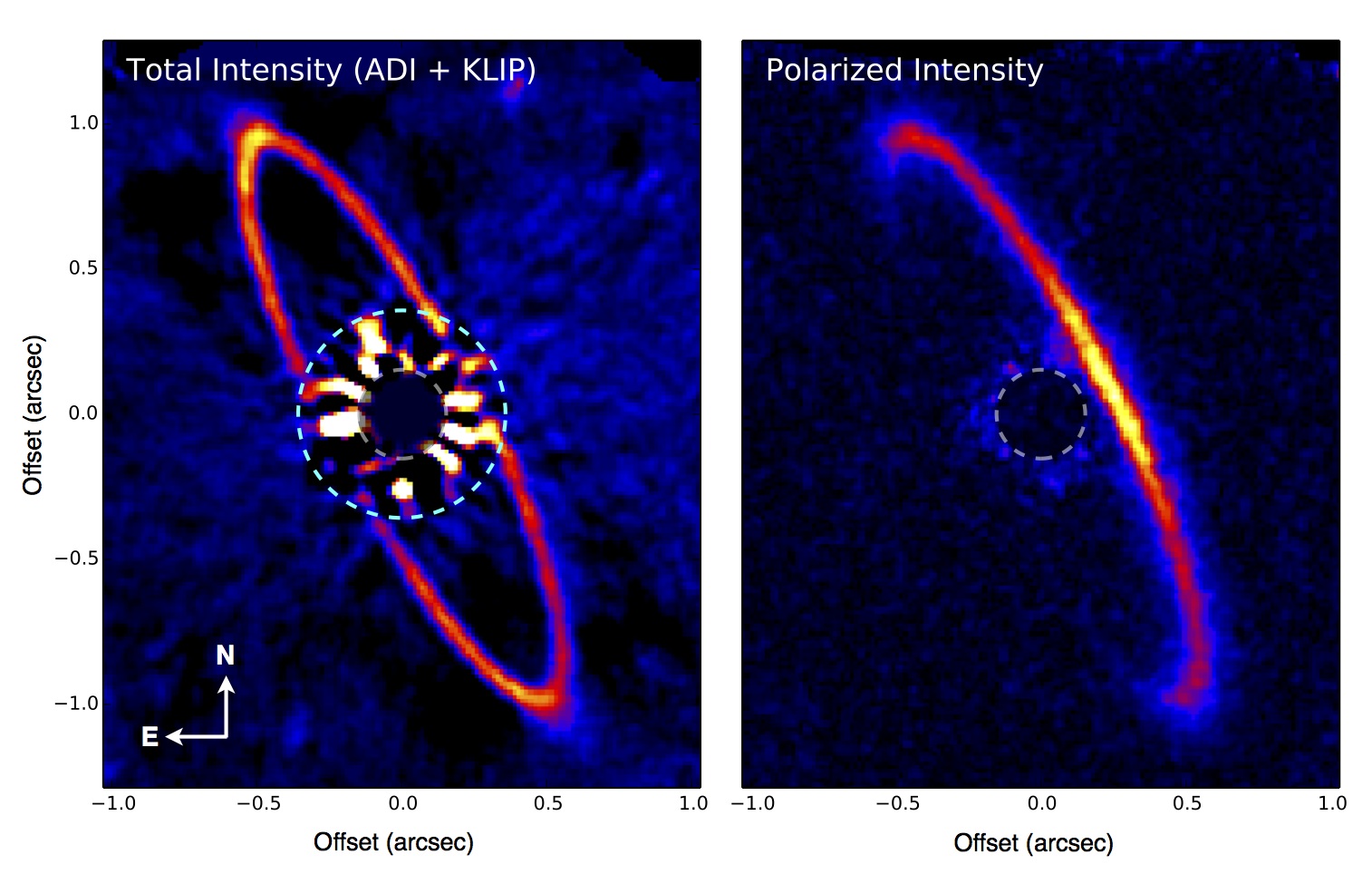
The field of exoplanetary research has seen a tremendous growth during the last decade, with the launch of space-based planet-hunting telescopes like the joint ESA/French Space Agency CoRoT and NASA’s Kepler telescopes, which have turned the discovery of new exoplanets around other stars from a historic occasion into a more or less routine procedure, as evidenced by the impressive tally of more than a thousand extrasolar worlds that have been confirmed by Kepler alone, as well as the thousands more that are still awaiting confirmation. Not to be outdone, a number of next-generation ground-based instruments that have also come on-line during the last couple of years promise to usher in a new era in exoplanetary science as well, allowing for the more detailed observation and characterisation of exoplanets around other stars through advanced direct imaging techniques. One such instrument, the Gemini Planet Imager, which is mounted on the 8.1-m Gemini South telescope in Cerro Pachón, Chile, has recently completed a year-long test and commissioning period, while capturing the images and spectra of previously discovered exoplanetary systems in unprecedented detail along the way, which are challenging several theoretical predictions regarding planetary formation.
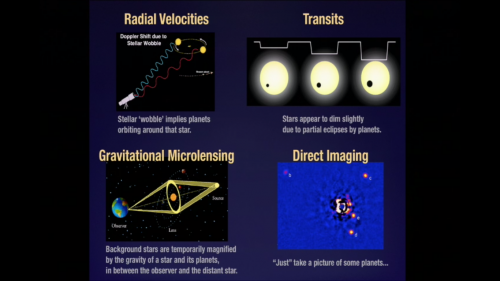
Apart from rough estimates concerning their mass, orbit, and size, little more is known about the bulk of exoplanets that have been found to date, due to the nature of the detection techniques that have been used for their discovery, namely the radial velocity and the transit method. More specifically, astronomers use the radial velocity method to detect any gentle, periodic “wobbles” in a star’s motion that might be caused by the gravitational tug of an unseen orbiting planet, while with the transit method they look for the dimming of a star’s light that would be caused by the passage of a planet across the star’s face. The closer a planet orbits its star, the greater its gravitational tug on it would be and the more times it would transit across the star’s disk, provided that it’s aligned properly with our line of sight here on Earth. Yet both of these are methods of in-direct detection, each with its own sets of advantages and limitations. For instance, radial velocity is best suited for finding massive planets that lie close to their host stars, because they cause a greater wobble to their host stars that can be detected more easily than that of less massive ones. Radial velocity has been mainly used for the detection of “hot Jupiters”—large and massive scorching gas giant planets that lie so close to their respective stars that their orbits are considerably smaller than that of Mercury’s in our Solar System. The downside of this technique is that it can’t detect planets that lie in wider orbits farther away from their host stars and can only be used to provide rough estimates for the planet’s minimum mass but not its size. The latter can be inferred with the use of the transit method, by measuring the depth of the characteristic dip in a star’s brightness as an exoplanet passes in front of its disk. Nevertheless, the transit method is also optimised for the discovery of planets with relatively short-period orbits, for the discovery of planets that are located far from their star would require many years or even decades of painstaking observations.
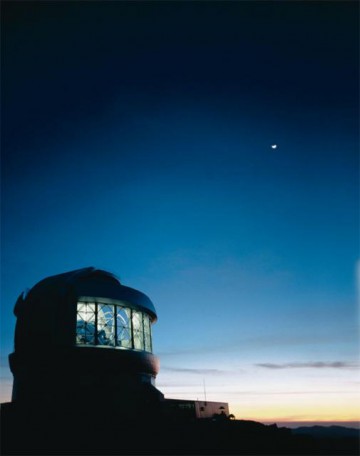
One of the best ways to detect and study new exoplanets in more detail is through direct telescopic observation and imaging. Yet, with present-day technology, such a prospect poses a much more formidable challenge than the mere exoplanet discovery itself. Lost in the overwhelming glare of its host star, the feeble reflected light from a nearby orbiting exoplanet, can be millions or even billions of times fainter. This intense starlight can saturate the field of view of even powerful space-based observatories like the Hubble Space Telescope, producing an excess glare that completely washes out the faint signatures of potential orbiting planets, making their detection extremely difficult. The situation becomes even worse for ground-based telescopes, which have to contend with the constant blurring and distorting effects of the Earth’s atmosphere, which can severely hamper observations. Nevertheless, the technical and engineering advances that have occurred in the construction of telescopes during the last couple of decades, as well as the implementation of novel observing techniques, have allowed astronomers to greatly overcome these challenges, opening the way for very detailed observations to be conducted with ground-based telescopes that were previously considered impossible. A key technology in this process is adaptive optics, which helps to cancel out in real-time the overall blur and distortion of astronomical images from the Earth’s atmosphere by continuously adjusting the shape of deformable mirrors on ground-based telescopes across the globe, thousands of times every second with millisecond accuracy, thus allowing for angular resolutions that rival those of space-based instruments.
Despite the many revolutionising contributions that adaptive optics technology have provided for observational astronomy, scientists were in need of instruments with a greater resolving power still, in order to be able to conduct any direct imaging observations of exoplanets from existing ground-based observatories. To that end, a consortium of 15 scientific institutions from the U.S. and Canada, including the Space Telescope Science Institute, NASA’s Jet Propulsion Laboratory and Ames Research Center, and the SETI Institute and the University of Montreal, set out in 2003 to conduct a feasibility study for the construction of an imaging system with an observing capability greater by at least a factor of 10 compared to that of similar systems on already operating telescopes. This ambitious project, called the “Gemini Planet Imager,” or GPI for short, started to materialise in 2005, when the multi-national Gemini Observatory Consortium, which was responsible for its funding and overall management, selected for implementation the GPI design proposal from the University of California, Berkeley. Following an extensive eight-year period of design, development, construction, and assembly of its various hardware components, the Gemini Planet Imager was finally integrated onto the Gemini South telescope in August 2013, in order to undergo a rigorous, year-long commissioning period.
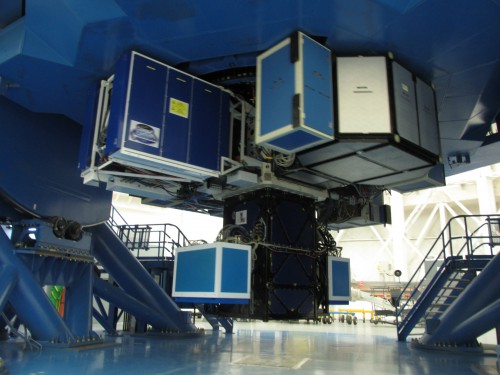
During this time, and having successfully achieved first light on Nov. 11, 2013, GPI’s science and engineering teams went through an extensive test and calibration process of the instrument’s full suite of subsystems, which include a set of two high-speed deformable adaptive optics mirrors, a state-of-the-art coronograph with a resolving power of 0.2 arcseconds for blocking out excess starlight, and an Integral Field Spectrograph, which operates in near-infrared wavelengths between 0.9 and 2.4 microns and can conduct detailed spectroscopic observations of exoplanets and circumstellar disks of unprecedented spatial resolution. GPI’s commissioning phase wasn’t all tests, however, with the instrument producing very detailed images of various celestial objects from its very first week of operations, which proved to be of high scientific value as well. An example of the Gemini Planet Imager’s superior capabilities was presented during a press conference at the recent 225th Meeting of the American Astronomical Society, which took place in Seattle, Wash., between 4 and 8 January, where Marshall Perrin, an associate astronomer from the Space Telescope Science Institute in Baltimore, Md., and leader of the Gemini Planet Imager Data Analysis Team, unveiled a pair of images and spectra of the very young planetary systems HR 8799 and HR 4796, whose planets are glowing brightly in infrared wavelengths while still radiating away the heat from their formation. The former, in particular, had been the first planetary system ever to be discovered through direct imaging, with the help of the adaptive optics systems on both the Gemini North telescope and the 10-m Keck telescope on Mauna Kea in Hawaii, which detected three massive gas giant planets in very wide orbits from their host star. Subsequent observations in 2010 also revealed the existence of a fourth planet, making HR 8799 one of the very few systems whose planets were located very far from their star.
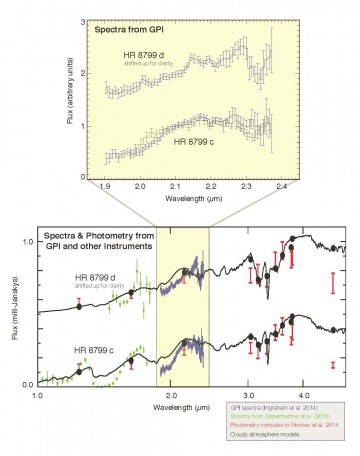
Even though these first images and spectra of the HR 8799 system, which had been taken with the previous generation Near-Infrared Coronagraphic Imager, or NICI, on the Gemini North telescope, were a feat of engineering, their quality was nevertheless greatly surpassed by those taken with the Gemini Planet Imager. More specifically, the new GPI spectroscopic observations revealed that all four planets in the HR 8799 surprisingly lacked the strong methane absorption lines that are found on the spectra of the gas giant planets in our own Solar System and are also thought to be quite common in low-mass brown dwarfs and young, massive gas giant planets like those of HR 8799. Furthermore, the spectra for two of the planets around HR 8799 exhibited some stark differences, indicating that, despite being members of the same planetary system while having the same age and similar color properties, these two planets must nevertheless have very different compositions. “Current atmospheric models of exoplanets cannot fully explain the subtle differences in color that GPI has revealed. We infer that it may be differences in the coverage of the clouds or their composition.” says Patrick Ingraham, a Post-Doctoral Scholar at Stanford University and leader of the team that studied the new GPI observations of HR 8799.
The images of HR 4796A proved to be of similar value to astronomers as well, providing intriguing details of the massive circumstellar dust disk that surrounds the young, 10-million-year-old star, which is located about 200 light-years from Earth. Previous observations had indicated that the structure and overall longevity of the dust ring is influenced by the presence of perturbing planets that are in the late stages of formation. Astronomers utilised GPI’s ability to conduct high-sensitivity polarimetry, which is a technique that takes advantage of an intrinsic feature of light waves, called polarization. More specifically, when a star’s light is reflected or scattered by the molecules in a planet’s atmosphere or the dust grains of circumstellar disks, its constituent light waves are aligned, or polarized, parallel along the direction of motion. By measuring this polarization with very precise instruments, astronomers can detect the faint light being reflected from a planet, among the extremely bright glare of unpolarized starlight. By using this technique, the GPI was able to image the entire circumference of the circumstellar disk around HR 4796A for the first time in unprecedented detail, which showed that its structure is much more opaque and dense than previously thought, somewhat contradicting theoretical predictions regarding the stability and dynamics of circumstellar disks in general. “GPI not only sees the disk more clearly than previous instruments, it can also measure how polarized its light appears, which has proven crucial in understanding its physical properties,” says Perrin. “These data taken during GPI commissioning show how exquisitely well its polarization mode works for studying disks. Such observations are critical in advancing our understanding of all types and sizes of planetary systems – and ultimately how unique our own solar system might be.” Exoplanetary systems aside, the Gemini Planet Imager conducted observations of several celestial bodies in our own Solar System as well, including Neptune, Jupiter’s moon Europa, and Saturn’s moon Titan. These allowed astronomers to study how the surface brightness on these objects changed in different wavelengths, providing useful insights on the study of similar color variations on distant exoplanets, which could potentially help scientists to better determine their chemical composition and internal structure.
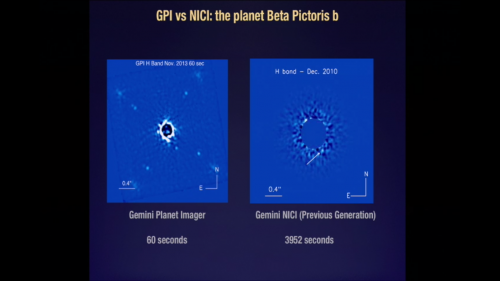
Now, with the commissioning of the Gemini Planet Imager being successfully completed in November 2014, astronomers have begun an observing campaign called the Gemini Planet Imager Exoplanet Survey, or GPIES, with the goal of studying 600 nearby stars of different spectral types, for a total of 890 hours over the next three years. The campaign’s primary objective is the discovery and detailed spectral characterization of Jovian-type planets in long-period orbits ranging between 5 and 40 astronomical units from their host stars, similar to the orbits of the gas giants in our own Solar System. Even though GPI won’t be able to directly image small, terrestrial worlds in Earth-type orbits, it nevertheless represents the next important step in that direction. “The entire exoplanet community is excited for GPI to usher in a whole new era of planet finding,” says Sara Seager, a professor of planetary science and physics at the Massachusetts Institute of Technology and a leading expert on exoplanets. “Each exoplanet detection technique has its heyday. First it was the radial velocity technique (ground-based planet searches that started the whole field). Second it was the transit technique (namely Kepler). Now, it is the ‘direct imaging’ planet-finding technique’s turn to make waves.”
The success and revolutionary engineering advances achieved by ground-based instruments like the Gemini Planet Imager could potentially one day be implemented into the designs of future space-based observatories, like the ATLAST 8 to 16-m space telescope concept, which has been proposed by NASA, that could prove to be the key for finally obtaining that long-sought image of an alien blue-dot, serenely orbiting at some distant corner of the Milky Way galaxy. Even though this prospect seems beyond the capabilities of today, it could well be tomorrow’s reality. “A telescope bigger than anything that exists on the ground right now, launched into space. It’s crazy, right?” commented Perrin, during a public lecture at the Space Telescope Science Institute. “But it was crazy to think about putting a 2.5-m telescope in space. It was crazy to think about having astronauts go up there and fix it and think about the James Webb Space Telescope with its giant unfolding sunshield. So, you can do crazy things when you are patient.”
As the saying goes, “patience is a virtue.” If that is the case, then our patience and perseverance in regards to space exploration and scientific research could help to answer some of the most fundamental questions of human existence.
Want to keep up-to-date with all things space? Be sure to “Like” AmericaSpace on Facebook and follow us on Twitter: @AmericaSpace